Scientists have coined a new type of porous material as a “cage of cages,” owing to its unique molecular structure capable of trapping carbon dioxide and other potent greenhouse gases.
Developed through collaboration between researchers in the UK and China, the material undergoes a two-step synthesis process. Reactions assemble triangular prism building blocks into larger, more symmetrical tetrahedral cages, resulting in the first molecular structure of its kind, as claimed by the team.
With an abundance of polar molecules, the resulting material attracts and retains greenhouse gases like carbon dioxide (CO2) with high affinity. Notably, it exhibits excellent stability in water, a crucial feature for its potential application in capturing carbon from wet or humid gas streams in industrial settings.

Marc Little, a materials scientist at Heriot-Watt University in Edinburgh and senior author of the study, describes the discovery as “exciting,” emphasizing the need for novel porous materials to address pressing societal challenges such as greenhouse gas capture and storage.
Although not yet tested at scale, laboratory experiments demonstrate the material’s high uptake of sulfur hexafluoride (SF6), identified by the Intergovernmental Panel on Climate Change as the most potent greenhouse gas. While CO2 remains in the atmosphere for 5–200 years, SF6 can persist for 800 to 3,200 years, with a global warming potential of over 23,500 times that of CO2 over a 100-year period.
Efforts to extract large amounts of CO2 and SF6 from the atmosphere or prevent their release are essential to mitigate climate change. However, existing carbon removal strategies, mainly relying on natural processes like tree and soil absorption, fall short of the necessary scale. Direct air capture technologies, which employ porous materials to absorb CO2 from the air, offer promise but currently contribute only a fraction of needed carbon removal.
Developing materials like the newly discovered cage-like structure could enhance direct air capture efficiency and contribute to climate change mitigation efforts. However, the urgency of the climate crisis demands rapid reduction of greenhouse gas emissions in addition to technological innovations.
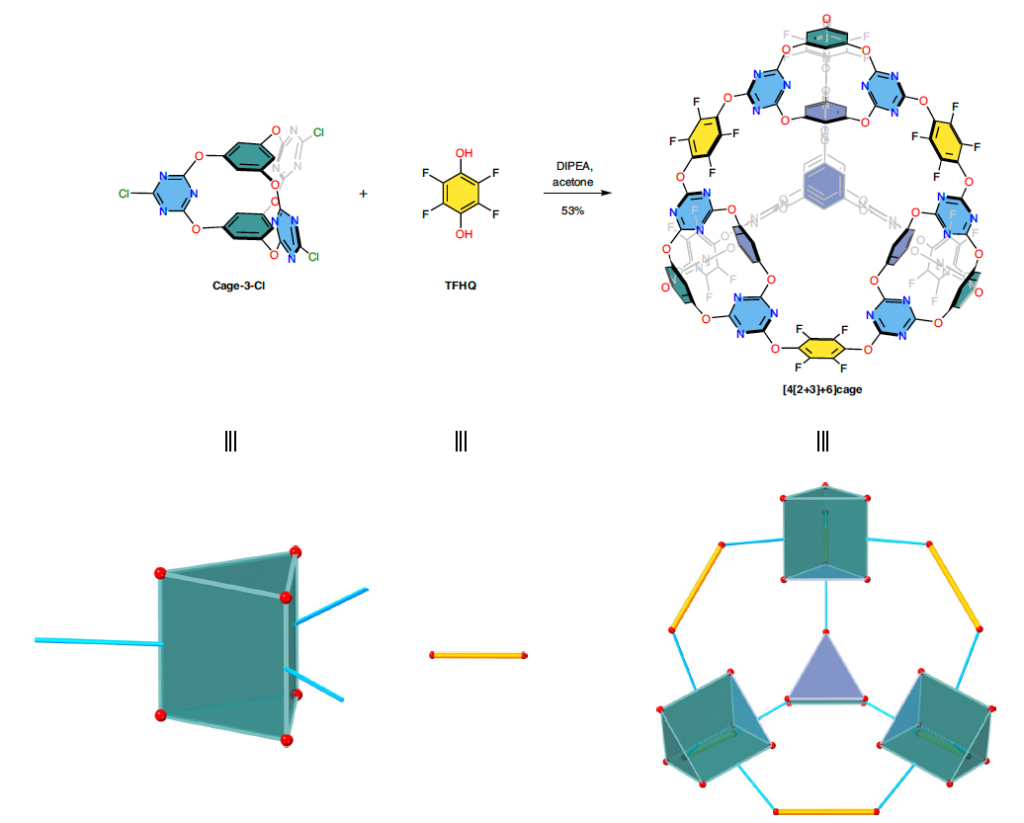
The material’s complex structural design posed challenges in its synthesis, despite the self-assembly of precursor molecules. Supramolecular self-assembly, a strategy utilized in its creation, requires careful optimization of reaction conditions due to the intricate molecular interactions involved.
Simulations were employed to predict the assembly of precursor molecules into the porous material, considering factors such as geometry, chemical stability, and rigidity. In addition to greenhouse gas absorption, the material shows potential for removing other airborne pollutants, such as volatile organic compounds, from the atmosphere, offering broader environmental benefits.
The study, published in Nature Synthesis, marks a significant step towards unlocking diverse applications for this innovative material in addressing environmental challenges.